Organoid technology can be traced back to early dissociation-reaggregation experiments. For example, in 1907, biologist Henry Van Peters Wilson, PhD, showed that dissociated sponge cells can self-organize into masses that turn into complete sponges.1 Subsequent exploration of the self-organization principle in cell biology, in combination with advances in cell culture, eventually led to true organoids, three-dimensional structures composed of various sorts of cells that together mimic an organ.
Today, organoids can be derived from pluripotent or tissue-resident stem cells or progenitor or differentiated cells from healthy or diseased tissues, and there are various organoid engineering strategies that support organoid culture and growth, proliferation, differentiation, and maturation.2 In addition, as this article emphasizes, the production of organoids is being carried out at ever greater scale, opening up a range of applications.
Increasing automation
Not too many years ago, even making an organoid proved very challenging, but that’s changing. “Lately, I have seen more papers and technologies figuring out how to make organoid culture and screening more accessible and more automated,” says Hilary Sherman, senior applications scientist, Corning Life Sciences. “This is going to be really important to take organoids beyond basic research and drive more widespread adoption in more high-throughput environments.”
Organoids can be made in various ways. One way is to grow cells on domes of extracellular basement membrane (ECM), for example, on Corning Matrigel Matrix attached to cell culture plates. “Matrigel matrix is a popular scaffold choice to support cell expansion in organoid cultures because it mimics the mechanical and chemical properties of the in vivo ECM and provides signaling cues via basement-membrane ligands involved in cell attachment and survival,” Sherman explains.
At room temperature, though, the Matrigel matrix can polymerize and “therefore can be challenging to use with the instrumentation,” Sherman says. “New protocols with automated platforms, like the Corning Matribot bioprinter, have temperature control that can keep Corning Matrigel matrix at an optimal temperature to allow it to be properly dispensed for organoid models and other applications.”
Plus, innovative organoid-growing methods might be particularly amenable to automation. As one example, Sherman points to recent work by scientists at Genentech. They developed “an entirely new method for generating Matrigel matrix organoid droplets suspended in media,” she points out. “This could be a game changer for automating organoid culture.” When the scientists at Genentech made their organoids, they employed automation to dispense an organoid/Corning Matrigel matrix suspension into prewarmed media.3
Still, much more work lies ahead to widely automate the production of organoids, but Sherman is optimistic. As she puts it: “I expect there to be further advances in making organoid culture easier, more reproducible, and higher in throughput—making it more accessible across many applications.”
Making organoids by the millions
In some cases, it’s already possible to scale up the production of organoids. In late 2022, Molecular Devices gained that capability through the acquisition of Cellesce, a company that specialized in large-scale organoid production. “We focus primarily on adult stem cell–derived organoids,” says Vicky Marsh Durban, PhD, director of custom organoid services, Molecular Devices, “but our platform technology is applicable to any type of organoid, as long as it grows in a gel matrix, because our bioreactor processes are optimized for growing organoids in gel matrices rather than in suspension.”

With this platform, Molecular Devices can produce up to six million organoids in a single batch, and the company’s next-generation platform will scale up production even more. The potential scale depends on the model. If the cells grow particularly densely or quickly, the scale can be increased even more.
“We’re working toward the theme of industrializing organoids,” Durban relates. “We want this amazing technology to expand from the academic space and reach the industrial space, too. We’re doing as much as we can to enable customers to start using organoids in particularly high-throughput settings where they’re going to get the most impact, the most value out of these complex models.”
To industrialize organoids, though, scale is not enough. “The biggest thing that we’ve kept in mind is really getting the scale without destroying the models,” Durban says. “The models are inherently quite sensitive and are more complex than the standard two-dimensional models.” Ensuring that so many organoids maintain the desired features depends on quality control.
“This is actually something that we’re still working on developing because there’s no precedent with this,” Durban notes. The company applies a range of standard tests, such as cell line sterility, but additional quality control often depends on the project and the customer’s required testing. As Durban explains, “We work quite closely with customers at the start of a collaboration to develop what the quality control package needs to look like.” Through Molecular Devices’ 3D Ready Organoid Expansion Service,4 a customer can receive a shipment of custom organoids in six months, depending on the type of organoids, the required scale, the endpoint assays, and so on.
Tonsil organoids
In 2021, scientists led by Mark M. Davis, PhD, professor of microbiology and immunology at Stanford University, reported that organoids developed from human tonsils can be used to study adaptive immunity.5 Based on that work, Charles River Laboratories has been optimizing the tonsil organoids “to understand how we might use them to further drug discovery,” says Louise Brackenbury, PhD, science director, advanced modalities, Charles River Laboratories.
After a routine tonsillectomy, the tonsils are immediately processed to single-cell suspensions, and the tonsil mononuclear cells (TMNCs) can be cultured at high density. This results in “defined structures reminiscent of germinal centers, located within secondary lymphoid tissue, such as lymph nodes or tonsils,” Brackenbury explains. “These structures better support B-cell responses by providing stromal populations as well as follicular helper T cells.” He adds that so far as is known, they are the best models for investigating human naive and memory B-cell function.
Vaccine responses in the human population vary significantly from person to person. Mimicking this, B-cell responses vary significantly in TMNC-developed structures between donors. “Donor-to-donor variability can be overcome by screening larger numbers of donors per test condition,” Brackenbury says. “Typically, we would suggest running three donors per test condition, whereas for the tonsil-organoid assay, we would suggest 5 to 10 donors.”
Although the tonsil-organoid system’s complexity and range of data collected makes high-throughput applications challenging, immunologist Lisa Wagar, PhD, of the University of California, Irvine, and her colleagues recently adapted this system to a 96-well format.6
Brackenbury says that overall, this system can be used for drug discovery and for studies of immune system function and disease pathogenesis, particularly in autoimmune disease. “We have already been using this system to initially optimize memory B-cell responses to vaccine antigens, such as influenza vaccine antigens,” she remarks. “And we are developing the ability to use it for immunogenicity screening of naive antigens and to evaluate adjuvants.”
For Charles River Laboratories, these applications are just the start. “Tonsil organoids are agnostic to therapeutic modality,” Brackenbury points out. “We have used the platform to assess immunogenicity of recombinant proteins, plasmid DNA expression systems, and inactivated viruses. We are also exploring how organ-on-chip (OOC) technologies, such as the OOC platform developed by Emulate, could help us explore therapeutic efficacy in a range of tissue-specific models in the presence of primary immune cells. The options are almost endless!”
Putting OOC technologies to the test
With OOC technologies, organoids can serve as the source of cells for making an organ-chip, which is essentially a three-dimensional culture system that closely mimics the natural microenvironment of the modeled organ. Examples include the Emulate Colon-Intestine Chip and Duodenum Intestine-Chip. “The way in which organ-chips recapitulate human tissue makes them well suited to various preclinical applications, particularly preclinical toxicity testing,” says Lorna Ewart, PhD, chief scientific officer, Emulate.
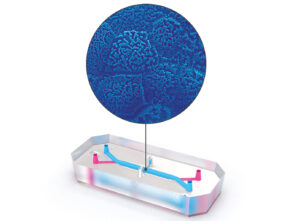
Despite the potential of organ-chips, the technology faces some significant challenges. “The first challenge is related to the need for industry-wide qualification criteria,” Ewart says. Fortunately, the International Consortium for Innovation and Quality in Pharmaceutical Development has created a roadmap for system qualification. Another challenge, Ewart points out, is the need to “take a hard look at animal models and collectively move toward a future in which drugs are developed using the best model systems, not just the traditional systems.”
Moving organ-chips ahead, though, depends largely on successful examples. In late 2022, Emulate reported on the predictive validity of its Liver-Chip in preclinical toxicology. The study assessed 870 Liver-Chips across 27 known hepatoxic drugs. The chip correctly identified 87% of the tested drugs that caused drug-induced liver injury in patients despite passing animal-testing evaluations. Plus, the Emulate human Liver-Chip did not falsely identify any drugs as toxic.
From automation and OOC technologies through tonsil cells and tons of organoids, these structures are just getting started as commercial-scale tools for healthcare research and the development of new treatments.
References
- Wilson HV. A new method by which sponges may be artificially reared. Science 1907; 25(649): 912–915.
- Zhao Z, Chen X, Dowbaj AM, et al. Organoids. Nat. Rev. Methods Primers 2022; 2: 94.
- Co JY, Klein JA, Kang S, Homan KA. Suspended hydrogel culture as a method to scale up intestinal organoids. Sci. Rep. 2023; 13: 10412.
- Molecular Devices. 3D ready organoid expansion service.
- Wagar LE, Salahudeen A, Constantz CM, et al. Modeling human adaptive immune responses with tonsil organoids. Nat. Med. 2021; 27: 125–135.
- Kastenschmidt JM, Sureshchandra S, Jain A, et al. Influenza vaccine format mediates distinct cellular and antibody responses in human immune organoids. Immunity 2023; 56: 1910–1926..