The transfer of human microbiota to mice has successfully transplanted phenotypes of obesity, depression, Parkinson’s disease, schizophrenia, and multiple sclerosis. Now, we can add autism spectrum disorder (ASD) to the list. A new study suggests that the gut microbiome and its metabolites contribute to hallmark ASD behaviors and associated neuronal activity and gene expression.
The stage was set in 2013, when the group of Jeffrey I. Gordon, MD, director of the Center for Genome Sciences and Systems Biology at Washington University School of Medicine in St. Louis, published the seminal paper, “Gut microbiota from twins discordant for obesity modulate metabolism in mice.” This work transferred fecal microbiota from adult female twin pairs discordant for obesity to illustrate that obesity phenotypes could be transplanted from humans to mice.
The data are “very exciting” asserts Emeran Mayer, MD, director of the G. Oppenheimer Center for Neurobiology of Stress and Resilience (CNSR) at the University of California, Los Angeles, who was not involved in the work. Mayer tells GEN that “this is the first study that confirmed the long-held, but unproven hypothesis that alterations in the gut microbiome (including neuroactive metabolites) play an important role in causing some of the characteristic ASD behaviors in mice.”
The paper, “Human Gut Microbiota from Autism Spectrum Disorder Promote Behavioral Symptoms in Mice” is published today in Cell.
The mouse behavior tests—tests that mimic the same behaviors that are associated with ASD in humans—laid the foundation for the work. For example, repetitive behavior that, in mice, results in burying more marbles in their cage over a certain period of time. Or, the amount of time spent socializing in a cage with another mouse. Although Gil Sharon, PhD, a post-doc in the Mazmanian lab and first author on the paper, notes that these tests are “not the same” as human phenotypes, he asserts that they are a good proxy for sociability or a fixation. He notes that one limitation with studying autism is that “we don’t really have any biomarkers.” In humans, he notes, the disorder is defined by behaviors which makes the behavioral assays important models to rely on. He adds that uncovering the difference between these mouse assays and human behavior will take “years of research.”

The mice used in these tests are the offspring of one parent mouse who has received the ASD fecal microbiome and one parent who received the TD microbiome. Mazmanian notes that they used the offspring to capture the prenatal developmental window and the exposure of the fetus as opposed to colonizing after weaning. This was driven, says Mazmanian, on the well-accepted belief in the ASD community that “a lot happens prenatally during development, in utero.”
Although Mazmanian does not call the humanized microbiome mice an “ASD mouse model,” he notes that this type of mouse model is “powerful.” One of the reasons for this is because the microbiome in a mouse coming from a commercial vendor is only about 5% similar to the human microbiome. So, improving on existing mouse models and getting them closer to the human condition is something that they hope their method can achieve.
Digging deeper
Based on the behavioral differences, Sharon notes, they wanted to determine the composition of the gut microbiota and neuroactive metabolites of the mice that were altered. The researchers measured the bacteria—both what went in and what came out—carefully assessing the level of engraftment of the bacteria. Mazmanian notes that their understanding of what bacteria constituted the samples is “quite comprehensive” because they performed shotgun sequencing in addition to analyzing by 16S rRNA gene sequencing. This rigorous approach allowed them to delineate species and strains from each other, offering more robust characterizations that could be used to infer pathway analysis.
With the help of the research group of Rob Knight, PhD, founding director of the Center for Microbiome Innovation and professor of pediatrics and computer science & engineering at the University of California, San Diego, metagenomic analysis identified key metabolites that may be modulating the behaviors and which bacteria contributed to the presence of those metabolites. They focused in on two metabolites that were depleted in the ASD transplanted mice, 5-aminovaleric acid (5AV) and taurine, to investigate further.
To do this, the researchers turned to a genetic mouse model of ASD, the BTBR T+tf/J model. In these mice, 5AV and taurine could alleviate some of the autistic-like behaviors. For example, the mice buried less marbles and doubled the amount of time they spent socializing. Also using the BTBR mouse model, the team performed electrophysiology to uncover that 5AV decreased the excitability of the mouse neurons—creating a balance between excitation and inhibition.
Knight tells GEN that “this is the first step towards figuring out which specific microbes cause these phenotypes, and how we can stop them.” Together with the recent open-label human fecal microbiota transplant trial in Arizona, Knight notes, these data “vindicate the long-held but long-disreputable idea that microbes contribute to autism in humans, rather than simply changing in response to autism.” He adds that the ability to transplant human phenotypes into mice via the microbiome provides an immensely powerful platform for dissecting the specific microbes, genes, and pathways that contribute to disease.
A big surprise
Mazmanian tells GEN that “the biggest surprise” of the results was that the ASD transplanted mice showed extensive alternative splicing of genes. This unexpected finding was made possible through their collaboration with Dan Geschwind, MD, PhD, at University of California, Los Angeles, an expert in the alternative splicing in ASD brains. They uncovered that the microbiome induces alternative splicing events in genes that, in some cases, are similarly spliced to those in the ASD brain—a result that Mazmanian finds “mind-blowing.”
One other paper to date shows a link between the microbiome and alternative splicing. The study, “Social interaction-induced activation of RNA splicing in the amygdala of microbiome-deficient mice” was published last year by the group of John Cryan, PhD, professor & chair of the department of anatomy & neuroscience at the University College Cork, Ireland. The researchers compared germ-free (GF) and ex-GF mice (GF mice that had been colonized with bacteria after weaning). GF mice tend to socialize less than mice born normally. When they looked at the reasons behind the social impairment of the GF mice and the gene expression in the brain, they found a different pattern of gene expression in the amygdala of their brain. When they asked what was the splicing profile of the amygdala, with or without social behavior, they could see splicing events that were a result of the microbiome status (GF versus ex-GF) and socialized versus non-socialized.
Cryan’s paper, notes Sharon, uses a very different model from the current study. Sharon analyzed the gene expression at 45 days after birth, before any social stimulation. What are the implications of microbiome induced alternative splicing? Sharon notes that there is “a very big question mark here.” But, the possibility that this could be a potential mechanism by which microbes affect behavior is something that he finds “very intriguing” and assures GEN that he will keep working on it.
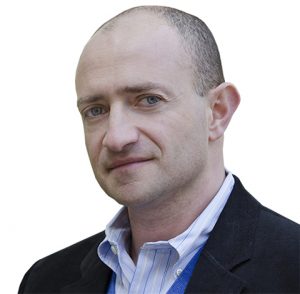
Mazmanian chooses his words carefully, cautious not to overhype these results, noting that they do not indicate that the microbiome is causal of ASD or that the microbiome is contributing in every single case. But, he tells GEN that “this work opens the possibility that the microbiome is not just a consequence of ASD, but, that it is a driver of symptoms” and that interventions are worth pursuing. He also notes that they did not exclude other mechanisms. For example, the role of the vagus nerve, immune response, hormones or neurotransmitters may be relevant in this system.
Even though the findings are exciting, there remain many limitations to extrapolate these findings to the treatment of ASD patients. “Of course, we must remember that mice are not humans,” notes Knight. Mayer agrees, noting that mouse models of neurological and psychiatric syndromes are notoriously limited and human ASD patients represent a heterogeneous group with different genetics and different underlying mechanisms. Mayer adds that children with ASD may have highly restricted eating preferences which almost certainly contribute to alterations in gut microbial metabolites. Sharon sees potential in using this system to study specific populations within ASD, and combining this model with genetic backgrounds, creating a powerful addition to the current ASD research landscape.