Proteins have been called the workers of the cell, and cells have been likened to factories. So, it was only a matter of time before proteins, protein pathways, and entire proteomes came to be seen through a workforce management or operations management perspective. Initially, this kind of management is descriptive. It takes inventories. It conducts time-and-motion studies. But these are mere preludes. The real goal is to overcome management’s central problem: designing and controlling processes.
The operations management theme is becoming more pronounced in proteomics now that mass spectrometry technology is moving beyond static inventories and attendance sheets. It is becoming more quantitative and dynamic, taking account of diverse protein characteristics and activities—stability, folding, and protein-protein interactions. It is also looking at proteins while allowing them to remain in suitably rich contexts. For example, mass spectrometry techniques are revealing how cells behave—how they alter their structural biology—in the living cell. Somewhat narrower select but still expansive) settings include kinomes, stress response pathways, and glycosylated proteins.
It should be emphasized that the proteomic work that is being enabled by mass spectrometry is not merely descriptive. It is also practical. Ultimately, the idea is to improve the operations that are performed by the little factories known as living cells. If there are personnel problems, they can, perhaps, be resolved—at the individual level or at the team level—potentially improving healthcare outcomes.
Identifying onset of illness
“We looked at biomarkers that mark early transition points from wellness to chronic disease,” says Leroy Hood, MD, PhD, senior vice president and chief science officer at Providence St. Joseph Health, and chief strategy officer and professor at the Institute for Systems Biology. “[We hope to use these biomarkers] in systems approaches to find disease-deterring methods and therapies before disease manifests itself.”
In 2014, Hood and colleagues started a pilot study in which 108 healthy individuals were longitudinally followed with genome and phenome analyses, including the analysis of 300–400 proteins, 150 chemical analytes, and 800–900 metabolites from the blood. The investigators also analyzed the gut microbiome of the study participants and collected information about digitally self-measured parameters, including sleep and physical activity.
In the initial nine-month study, the phenome analyses were conducted every three months. Subsequently, 5000 more individuals were recruited into a four-year study. During the larger, longer study, over 100 of the participants developed various diseases.
“We were able to look at each of these patients in detail,” recalls Hood. “To assess the transition from wellness to disease, we analyzed blood samples for approximately 1200 analytes to see if we could find any outliers.”
Ultimately, five striking outliers were found, including three proteins that are members of the Notch receptor pathway, one of the most frequently activated signaling pathways in cancer.
Hood and colleagues are using this approach in a million-person project that will continue over the next five years. It aims to create a shared context for genomic and phenomic findings as well as electronic medical records.
“The whole vision of how we look at disease is going to be utterly transformed,” declares Hood. “It will mean having metrics to measure the degree and the extent of wellness, to detect the transition that initiates disease, and to follow the increasingly complex amplifications of disease.”
This paradigm change promises to disrupt clinical practice. “This is similar to what happened with the genome project,” suggests Hood. “It took five years to convince the world that we should do it, and once it was done, it generated technologies and data on many organisms that transformed how we think about biology.”
Dissecting the kinome
The proteomic methods used to profile protein kinase inhibitors should facilitate the identification and characterization of specific inhibitors. Unfortunately, conventional methods have contributed to a “plague” of nonspecific inhibitors, complains Lee M. Graves, PhD, professor of pharmacology at the University of North Carolina at Chapel Hill select UNC). To help dispel the plague, Graves contributes to the development of refined proteomic methods.
For example, Graves and his UNC colleagues select including the recently retired Gary L. Johnson, PhD) developed a platform called multiplexed inhibitor beads select MIBs). With this platform, several kinase inhibitors may be immobilized on a Sepharose column in a specificity gradient, allowing kinases to be captured at different layers on the column and subsequently examined by quantitative mass spectrometry. Because this approach combines MIBs and mass spectrometry, it is called MIB/MS. It can be used to reveal how kinome dynamics change in response to inhibitors or cellular conditions, or upon the emergence of drug resistance.
“With our capture method, we can see 75–80% of the expressed kinome, but not all kinases bind to the beads,” admits Graves. “Sometimes, we need to reengineer the chemical material to broaden the scope of capture.”
In collaboration with Nathaniel J. Moorman, PhD, associate professor of microbiology and immunology at UNC, Graves used MIB/MS to quantitate perturbations in >240 kinases in cells infected with human cytomegalovirus and profile the time-dependent changes in the cellular kinome. Using the data and viral replication assays, Moorman and Graves identified three inhibitors of viral replication that were effective at doses that did not show cellular toxicity. One of these compounds, an inhibitor of the serine threonine kinase MELK, caused an over 3-log decrease in viral replication, indicating its potential as a novel antiviral drug.
“This approach led to a large initiative to look at the kinome response to Middle East respiratory syndrome,” notes Graves. “We also anticipate looking at other pathogens, including herpes simplex virus and coronavirus, to profile the response and see whether some of the changes that occur can be targeted as new antiviral approaches.” Graves is currently working with leading virologists at UNC, supported by the Eshelman School of Pharmacy, to complete this important work.
In another study, Graves collaborated with UNC’s Channing Der, PhD, and others on a project that used a flow cytometry–based assay to screen a library of >800 protein kinase inhibitors. The investigators identified compounds that promoted either the stability or the degradation of MYC in a KRAS-mutant pancreatic ductal adenocarcinoma cell line. For example, CDK9 was found to enhance MYC protein stability through a previously unknown KRAS-independent mechanism that involved the phosphorylation of a specific serine.
“There is a huge amount of interest in using this technology to explore understudied kinases because our approach is unbiased,” asserts Graves.
The concept of kinome reprogramming is of particular interest in Graves’ group. Several studies revealed kinase remodeling in a time-dependent manner, largely involving transcriptional changes or their downregulation or degradation. “The dynamic nature of the kinome probably prevents single inhibitors from being as effective as they would because the kinome remodels around them and escapes their inhibitory effect,” explains Graves.
One of the limitations in kinome profiling experiments is that low-abundance proteins may not generate sufficient numbers of peptides to provide statistical confidence. “But with some new separation techniques and methods of quantification, some of those problems become less and less limiting,” Graves maintains. “As the mass spectrometers become more sensitive, we are able to see more things than we were able to see before.”
Determining protein structures in living cells
“My lab is pursuing a lot of method development, and the major question we are asking is whether we can perform structural biology in cellular systems to provide information across the proteome,” says Lisa M. Jones, PhD, associate professor of pharmacological sciences at the University of Maryland School of Pharmacy. One of the techniques used in Jones’ lab is fast photochemical oxidation of proteins select FPOP union a mass spectrometry–based protein footprinting approach. It is proving to be valuable in structural proteomics studies.
“We try to get around purification by studying the proteins directly in their native cellular environment,” Jones points out.
FPOP relies on the irreversible labeling of exposed amino acid side chains by hydroxyl radicals. In this technique, a flowing solution of protein and hydrogen peroxide is irradiated by a laser, triggering the production of hydroxyl radicals that subsequently modify the protein. Jones and colleagues expanded FPOP and created in-cell FPOP select IC-FPOP union which allowed protein footprinting to be performed in live cells for the first time. The technique’s potential was demonstrated in successful studies of protein-protein interactions in Caenorhabditis elegans and several mammalian cell lines.
A more recent approach developed in Jones’ lab, Platform Incubator with movable XY stage select PIXY union replaced the single-flow system with a sterile incubator that incorporates not just a mobile stage, but also peristaltic pumps, as well as mirrors for guiding laser beams.
“It takes about 20 seconds to perform an experiment with this new platform, as compared to 10 minutes with our previous flow system,” asserts Jones. Generally, the time scale for the tertiary structure folding of proteins ranges from milliseconds to seconds, and powerfully positions PIXY to detect events in this range.
A challenge with these experiments is the vast amount of data that is generated. “Data analysis is definitely a bottleneck,” advises Jones. “A good software that not just searches the data but helps with quantitation would be very helpful.”
Gauging cellular responses to environmental cues
“For a long time, I have been interested in understanding how cells sense and respond to their environment,” says Jeffrey A. Lewis, PhD, assistant professor of biological sciences at the University of Arkansas. The cellular response to environmental factors has been traditionally studied at the transcriptional level, by capturing changes in RNA molecules over time. This approach predominated, in part, because RNA was deemed a reasonable proxy for protein. Also, RNA is much easier to quantitate.
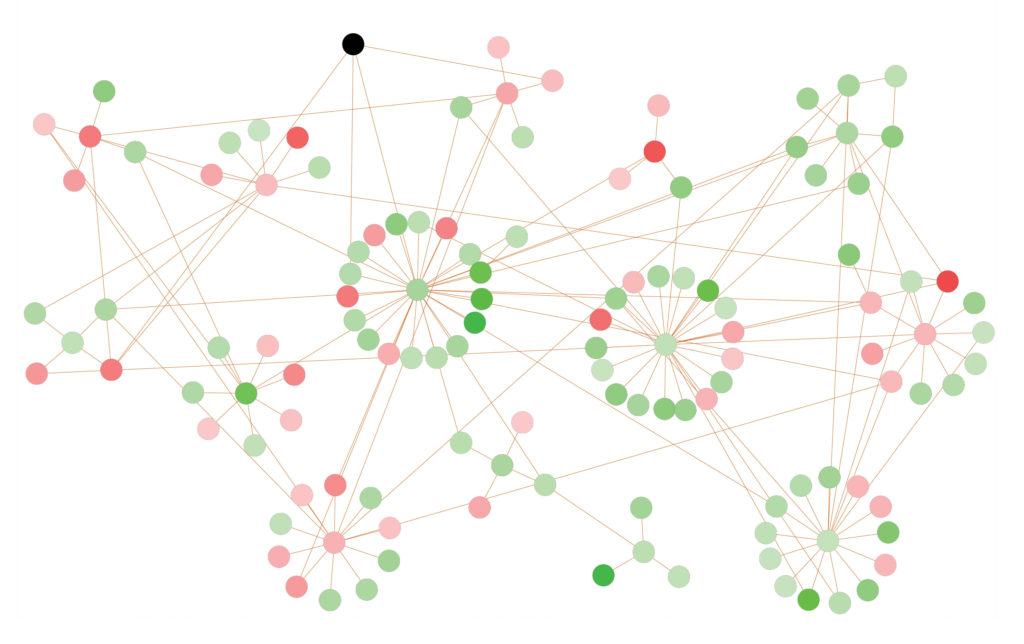
“With the availability of sensitive mass spectrometers, we are now able to accurately quantitate protein,” Lewis maintains. Protein quantitation, though not as good as RNA quantitation, is good enough, he continues, to allow investigators to “start looking at the ultimate mediators of environmental responses.”
A major effort in Lewis’ group is focusing on interrogating post-translational protein modifications during the classic heat shock response, a pathway that has been well studied at the transcriptional level in several organisms. “We used mass spectrometry to get a detailed view of an acute and dynamic measure of the stress response over time,” notes Lewis.
Using tandem mass tagging select TMT) triple-stage mass spectrometry select MS3 union scientists in Lewis’ lab examined the heat shock response in the budding yeast over nine time points, from five minutes to four hours after the heart shock. This approach quantitated >2000 proteins and revealed that their differential expression peaked approximately 90 minutes after the heat shock as compared to unstressed, control cells. The induced proteins could be organized into three clusters, and analyses of transcription factors that regulated the genes from each cluster indicated that early-induced gene were more likely to be the ones that directly respond to the heat shock.
One of the challenges in quantitative proteomics is that in complex biological samples, low-abundance species are masked by the high-abundance ones, making it very difficult to learn about proteins with low expression, which nevertheless fulfill important biological roles. “It is still a question whether the dynamic range of protein level changes is being compressed by the technology,” observes Lewis. This translates to a certain degree of uncertainty over how accurate fold-change calculations are. “We are doing pretty well with measuring relative abundance,” says Lewis, “but absolute abundance is a big challenge.”
Interrogating cell surface glycosylation
“Compared with many other types of protein modifications, the study of glycosylation has been more challenging,” states Ronghu Wu, PhD, associate professor of chemistry and biochemistry at Georgia Tech. Research in Wu’s lab focuses on protein glycosylation, including the development of new methods to examine its distribution and function in cells.
As one of the most frequent modifications, protein glycosylation is ubiquitously distributed in cells across species and is essential for a wide variety of biological processes in development, homeostasis, and disease. “The surfaces of cells are covered by glycans,” says Wu. “This happens in all species, even in bacteria.”
Almost all proteins on the cell surfaces are glycosylated, and aberrant glycosylation is linked to human disease, including malignant transformation, inflammatory responses, the immune escape of viruses, and certain genetic conditions. “Protein glycosylation,” Wu emphasizes, “is also very important for biomarker identification and drug discovery.”
A new method developed by Wu and colleagues enriches glycopeptides by conjugating benzoboroxole, a derivative of boronic acid, to a dendrimer. The dendrimer provides a powerful platform to conjugate many benzoboroxole molecules to the same dendrimer bead and offers structural flexibility to establish stronger synergistic interactions with several sugar residues within a glycan.
This approach is particularly powerful for detecting low-abundance glycopeptides, which can be masked by highly abundant species during routine biochemical and molecular analyses. Working with yeast cells, mouse brain tissues, and several human cell lines, scientists in Wu’s lab used this technology to site-specifically identify N– and O-glycoproteins including many low-abundance ones.
In a study that metabolically labeled and enriched surface glycoproteins with a sugar analog containing a small functional group, Wu and colleagues characterized over 2000 N-glycosylation sites from over 1000 glycoproteins in eight human cell types. This analysis showed that glycosylation sites are dramatically impacted by the secondary structure of proteins and are predominantly distributed in disordered protein regions.
Interpreting the functional significance of glycosylation has been challenging. Part of the difficulty is attributable to the existence of multiple mechanisms for glycosylation. In addition, the types of sugar residues, the linkages, and the sizes of glycans all contribute to a wide variety of glycosylation modifications across species. “Glycans are highly diverse,” Wu points out, “and the heterogeneity of glycans also makes glycopeptide enrichment much more challenging.”
Benchmark of SARS-CoV-2 Glycan Shield with Mass Spec Analysis
Scientists worldwide are working tirelessly to develop a vaccine for SARS-CoV-2 select the COVID-19 betacoronovirus union focusing on the humoral immune response to the spike select S) glycoprotein. Understanding the S protein’s glycosylation can reveal fundamental features of viral biology for use in the development and manufacturing of serological testing kits and potential vaccines.
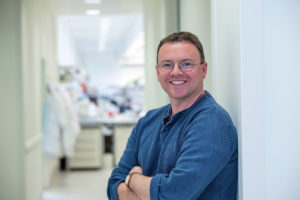
Max Crispin, PhD
“We used an Orbitrap™ mass spectrometer select from ThermoFisher) with advanced analysis software select from Protein Metrics) to characterize both the site-specific N-linked glycan composition and the degree of sequon occupancy on a soluble, native-like SARS-CoV-2 S protein,” says Max Crispin, PhD, a professor of glycobiology at the University of Southampton, U.K. “The sequon occupancy likely plays a role in immune evasion and occlusion of immunogenic proteins.”
According to Crispin, the team’s analysis1 mapped the glycan processing states across the trimeric viral spike and allowed the researchers to show how SARS-CoV-2 glycan processing differs from that of typical host glycans. The Byonic™ and Byologic® software suite from Protein Metrics enabled rapid characterization of the complex data and detailed comparison of site-specific glycosylation, he added.
The SARS-CoV-2 gene encodes 22 N-linked glycan sequons per protomer and utilizes this extensively glycosylated spike protein to bind to the host cell receptor. Notably, an array of host-derived glycans dominate the spike surface, each trimer displaying 66 N-linked glycosylation sites. This extensive glycosylation has important implications for vaccine design.
“We found that oligomannose levels—and hybrid-type glycan quantities—are significantly lower than what is observed on other viral glycoproteins. This suggests that SARS-CoV-2 S protein is less densely glycosylated, possessing less of a ‘glycan shield,’ which may be beneficial for the elicitation of potent neutralizing antibodies,” Crispin notes.
For each of the 22 glycan sites, the occupancy is nearly fully complete, suggesting that any epitopes shielded from the immune system on the virus will also likely be shielded on the immunogen.
“This kind of detailed site-specific glycosylation analysis, using mass spectrometry and advanced protein characterization software, is increasingly used to compare different immunogens and to target strategies for detection and vaccine development,” explains Crispin.
References